Automotive Electrification and Power Supply Design
Automotive and Electrification represent a significant advancement in automotive technology, offering a sustainable alternative to traditional internal combustion engine vehicles. Powered by electricity stored in batteries, Electric Vehicles (EVs) produce zero tailpipe emissions, which significantly reduces air pollution and greenhouse gas emissions. Additionally, EVs often feature lower operating and maintenance costs due to fewer moving parts. As renewable energy sources like solar and wind become more integrated into the grid, the environmental benefits of EVs will further increase, making them a pivotal component of a sustainable future in transportation. Automotive and Electrification refers to the integration of electric powertrains and components into vehicles – both EVs and Hybrid Vehicles. A hybrid powertrain integrates both internal combustion engine (ICE) and electric motor to achieve improved fuel efficiency, reduced emissions, and enhanced vehicle performance. Their powertrains come in various configurations, including mild hybrids, full hybrids, and plug-in hybrids, each with differing levels of electrification and complexity. In this article we look prominently into Automotive and Electrification, EVs, the power supply system and associated electronics.
EV Architecture
As the automotive industry moves towards electrification, understanding EV architecture is essential for grasping how these vehicles are designed to meet the demands of modern transportation, offering a cleaner, smarter, and more efficient driving experience.
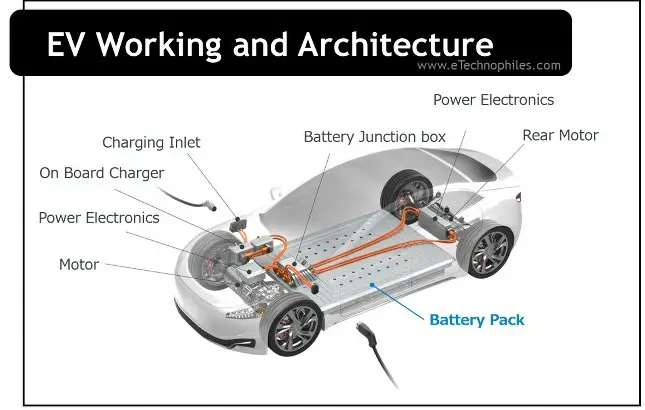
Source: eTechnophiles.com
The core components of EV architecture include the electric motor, battery pack, power electronics, thermal management system and transmission system. The EV architecture also encompasses advanced software and control systems, which enhance vehicle performance, safety, and connectivity. The electric motor, often an AC induction or permanent magnet motor, converts electrical energy from the battery into mechanical energy to drive the wheels. The battery pack, typically composed of lithium-ion cells, stores the electrical energy required to power the vehicle. Power electronics, such as inverters and converters, manage the flow of electricity between the battery, motor, and other vehicle systems. The transmission system in EVs is generally simpler, often using a single-speed gearbox due to the wide torque range of electric motors. Additional elements like thermal management systems ensure optimal operating temperatures for the battery and motor, enhancing performance and longevity.
Key Components in Automotive and Electrification
Electric Vehicles (EVs) integrate various electronic components to ensure efficient performance, safety, and comfort. At the core of an EV is the battery pack, which stores the electrical energy required to power the vehicle’s electric motor. Automotive and Electrification, particularly the power supply architecture, is designed to manage and distribute electrical energy efficiently across various vehicle systems, converting DC to AC power. This includes Electronic Control Unit (ECU), Battery Management System (BMS), On-Board Charger (OBC), DC-DC converter, and more. The architecture is designed with modularity, redundancy, and advanced control algorithms to enhance reliability, efficiency, and adaptability to future innovations like wireless charging and vehicle-to-everything (V2X) capabilities. Let’s look at the key components of an EV electrification and power supply architecture:
Electronic Control Unit (ECU): The Electronic Control Unit acts as a central control and coordination hub for ECUs of many subsystems including Engine control Unit, Battery Management System, Transmission Control, ABS, Electronic stability Control, etc. ECU utilize high-level commands and interfaces such as FSI (Fast Serial Interface), CAN, and LIN for communication between subsystems. Each subsystem contains MCU-powered application-specific control units responsible for process, control, and communication.
Battery Management System (BMS): The Battery Management System monitors and manages battery pack parameters including voltage, current, temperature, state of charge (SOC), and state of health (SOH). It employs coulomb counter circuits for precise charge estimation, ensuring safe operation by regulating charging and discharging cycles using active or passive cell balancing techniques.
Charging Distribution Unit: This system provides switchover between single-phase and three-phase charging through the onboard charger to DC fast and supercharging. It utilizes a contactor-based switchover design for high power transfer, incorporating high-performance contactor drive circuits (analog design) for fast response and quick isolation.
On-Board Charger (OBC): The On-Board Charger converts AC power from the grid to DC power to charge the battery, with an AC to DC stage that includes Power Factor Correction (PFC) and a DC-to-DC stage for traction battery compatibility. It supports bi-directional power transfer for Vehicle to Grid (V2G) applications and must accommodate various charging standards and power levels up to 22kW.
Traction Battery Pack: The EV traction battery is a rechargeable energy storage system that supplies power to the electric motor very quickly, providing high performance and rapid acceleration. Leading battery technologies include Lithium-Ion and Lithium-Ion Phosphate cell chemistry.
Traction Inverter: This component converts DC power from the traction battery to AC power for the electric motor. It must be highly efficient and capable of handling high power levels, utilizing high-power H-Bridge circuits with SiC MOSFETs and/or insulated-gate bipolar transistors (IGBTs) with high-precision gate driver circuitry for precise power flow to and from the electric motor.
Motor Control: The motor controller regulates the electric motor’s power delivery based on accelerator pedal input, ensuring precise control over speed and torque. It uses advanced power electronics to modulate electrical energy from the battery and microcontrollers to execute real-time performance adjustments through complex algorithms. Sensors, including position, current, and temperature sensors, provide continuous feedback, enabling optimal efficiency, performance, and thermal management. The controller also integrates fault detection and diagnostics to enhance reliability and safety, ensuring smooth, responsive, and efficient power delivery essential for the EV’s performance and drivability.
Traction Electric Motor/Generator: The main propulsion device in an electric car, the traction electric motor/generator converts electrical energy from the traction battery into mechanical energy. Commonly used systems include three-phase induction motors and DC motor systems, with resolver-based analog designs for closed-loop feedback control.
Auxiliary DC-DC Converter: This converter steps down the high-voltage battery power to lower voltages for auxiliary systems, such as 12V, 24V, and 48V. It is a key source for supplying power to vehicle electronics, lighting, infotainment systems, and other electrical systems, incorporating both isolated and non-isolated power supplies for different applications.
Signal Conditioning Circuitry: This circuitry collects data from multiple sensors and transmits it to various nodes throughout the system. Discrete analog solutions are implemented to ensure minimal data loss during transmission over longer distances.
Circuit Protection System: The circuit protection system provides path selection to different subsystems within the auxiliary power supply architecture. It includes electronically controlled fuses and protection mechanisms for real-time monitoring of current, voltage, and temperature, with fault detection and fuse actuation. Protection circuits also incorporate EMI/EMC filter designs compliant with ISO standards ISO 7637-2 and ISO 16750-2.
Vehicle Control Unit (VCU): The Vehicle Control Unit (VCU) in an EV is a centralized control system that coordinates the operation of various subsystems, such as the Battery Management System (BMS), motor controller, and thermal management system. It utilizes microcontrollers to execute complex software algorithms that manage and optimize the performance of these subsystems. Communication interfaces facilitate data exchange between the VCU and other vehicle components, ensuring seamless integration and coordination. By processing real-time data from various sensors and control units, the VCU enhances the overall efficiency, safety, and performance of the EV, providing a cohesive and responsive driving experience.
Design Requirements for Automotive and Electrification
Designing a power supply for an electric vehicle (EV) involves several key requirements to ensure performance, efficiency, and safety.
Electrical Requirements: Typical battery pack voltages range from 150V to 800V, with some high-performance models reaching up to 1000V. Low voltage system architectures (12V, 24V, or 48V) are used for auxiliary functions. The power supply must handle high currents, especially during acceleration (1100A and above) and fast charging. Components must be rated for peak current demands. High efficiency is critical to maximize the range and performance of the EV.
Thermal Management: Thermal management in EV systems is crucial for ensuring the optimal performance, safety, and longevity of key components such as battery packs, electric motors, and power electronics. Efficient thermal management systems utilize a combination of air and liquid cooling methods to maintain appropriate temperatures, preventing overheating and thermal degradation. Liquid cooling is particularly effective for high-performance applications, providing uniform temperature control. Additionally, advanced materials like thermal interface materials and phase change materials enhance heat dissipation. Integrated thermal management systems coordinate the cooling of batteries, motors, power electronics, and cabin climate control through sophisticated software. They use sophisticated software for real-time optimization based on driving conditions and ambient temperature.
Battery Cooling and Heating: This maintains optimal battery temperature to ensure efficiency, safety, and longevity. It utilizes air cooling, liquid cooling, and refrigerant cooling systems, and includes heating systems in cold climates to maintain performance.
Electric Motor and Power Electronics Cooling: Liquid cooling systems dissipate heat generated during operation. Heat sinks, thermal interface materials, and active cooling are used to manage heat in components like inverters and converters.
Safety and Protection: Overcurrent protection involves fuses, circuit breakers, and electronic protection circuits to prevent damage from overcurrent conditions. Overvoltage and under voltage protection safeguard components from voltage spikes and drops, ensuring stable operation. Electrical isolation between high-voltage and low-voltage systems is essential to prevent accidental shock and ensure safety. Fault detection and management systems detect and respond to faults, including short circuits, thermal runaway, and component failures. An emergency mode power supply and switchover systems provide control over the EV in fault events.
Environmental and Mechanical Considerations: Components must withstand the mechanical stresses of automotive environments, complying with standards such as ISO 16750-3 for mechanical loads. Waterproofing and dustproofing ensure components meet IP67 or higher ratings for protection against dust and water ingress. Designing for electromagnetic interference (EMI) and electromagnetic compatibility (EMC) prevents interference with other electronic systems, complying with standards like CISPR 25 and ISO 11452.
Automotive Standards for Automotive and Electrification
Several standards guide the design and safety of Automotive and Electrification in electric vehicles. These standards ensure interoperability, safety, and performance consistency across different EV models.
International Standards
ISO 26262: Functional Safety for Road Vehicles
ISO 26262 focuses on the safety of electrical and electronic systems within road vehicles. It introduces the Automotive Safety Integrity Level (ASIL), a risk classification system defined by the standard for the functional safety of road vehicles. The standard emphasizes risk assessment and mitigation strategies throughout the vehicle lifecycle to ensure safety.
IEC 61851: Electric Vehicle Conductive Charging System
IEC 61851 specifies the general requirements for conductive charging systems for electric vehicles. It covers various aspects such as charging modes, communication protocols, and safety requirements to ensure reliable and safe operation during the charging process.
IEC 62196: Plugs, Socket-Outlets, Vehicle Connectors, and Vehicle Inlets
IEC 62196 defines the requirements for connectors and inlets used for conductive charging of electric vehicles. This standard ensures compatibility and safety in the physical connections between the charging infrastructure & the vehicle.
Regional Standards
SAE J3400: North American Charging Standard (NACS)
SAE J3400, an EV charging connector system developed by Tesla Inc., supports both AC charging and DC fast/supercharging. This standard is tailored to meet the specific requirements of the North American market, providing a unified approach to EV charging.
SAE J1772: Combined Charging System (CCS)
SAE J1772 specifies the physical, electrical, and performance requirements for the charging coupler. Widely adopted in Europe and Japan, this standard ensures compatibility and performance across different regions, facilitating a seamless charging experience for EV users.
GB/T 20234: Connection Set for Conductive Charging of Electric Vehicles
GB/T 20234 is China’s standard for EV charging connectors, focusing on safety and compatibility. This standard ensures that EV charging infrastructure in China meets stringent safety requirements and is compatible with various EV models.
CHAdeMO: Quick Charging Standard for Electric Vehicles
CHAdeMO defines the requirements for high-power DC fast charging. This standard is used internationally to provide a reliable and efficient method for quickly charging electric vehicles, ensuring high performance and compatibility across different EV models.
Other Relevant Standards
ISO 15118: Road Vehicles – Vehicle-to-Grid Communication Interface
ISO 15118 specifies the communication interface between electric vehicles and the electric grid. This standard supports the integration of EVs into the grid, enabling functions such as vehicle-to-grid (V2G) communication, which allows EVs to return stored energy to the grid.
ISO 21434: Road Vehicles – Cybersecurity Engineering
ISO 21434 addresses the cybersecurity aspects of automotive systems, which is crucial for modern electric vehicles with interconnected systems. This standard provides guidelines to ensure the protection of EVs from cyber threats, ensuring the safety and security of both the vehicle and its occupants.
Design Best Practices
Designing efficient and robust Automotive Electrification and power supply systems is crucial for ensuring the performance, safety, and reliability of modern EVs. A holistic approach is necessary to ensure that EVs meet the demands of modern transportation, providing users with a seamless and dependable driving experience.
Modular Design
Modular design stands as a foundation of effective EV engineering, offering advantages in upgrades, maintenance, and scalability. By compartmentalizing components, this approach facilitates the seamless replacement of individual modules without necessitating extensive system overhauls. Such flexibility ensures streamlined maintenance procedures and enables future-proofing against evolving technological advancements.
Optimized Battery Management System (BMS)
An optimized Battery Management System (BMS) is essential for monitoring and managing the battery pack. High-precision sensors should be used to monitor voltage, current, and temperature of individual cells as well as the entire pack. Implementing active or passive cell balancing maintains uniform charge levels across cells, enhancing battery life and performance. Additionally, incorporating multiple layers of safety protocols and redundant circuits helps detect and mitigate overcharge, over-discharge, and thermal runaway scenarios.
Efficient Power Electronics
Power electronics, which convert and control the flow of electrical energy in an EV, require the use of high-efficiency DC-DC converters and inverters to minimize energy losses. Effective thermal management systems should be designed to dissipate heat generated by power electronics, ensuring longevity and reliability. Ensuring that electromagnetic interference (EMI) and electromagnetic compatibility (EMC) standards are met is crucial to prevent disruptions to vehicle electronics.
Reliable Charging Systems
Designing charging systems that support various charging standards, such as CCS, CHAdeMO, and Tesla Supercharger, ensures broader compatibility. Fast-charging capabilities should be implemented while ensuring that the battery’s health and safety are not compromised. Integrating smart charging features optimizes charging time and cost and incorporating technologies like vehicle-to-grid (V2G) technology allows energy flow back to the grid.
Robust Electrical Architecture
The electrical architecture interconnects all the electrical and electronic components in an EV. Designing redundant wiring and communication pathways ensures reliability in case of a single point of failure. Using a modular approach facilitates ease of maintenance and scalability, allowing for future upgrades and component replacements. Implementing strict safety protocols and insulation for high-voltage components protects users and service personnel.
Advanced Thermal Management
Effective thermal management is essential for maintaining optimal operating temperatures in EVs. Integrated cooling systems for the battery pack, power electronics, and electric motor ensure efficient heat dissipation. Incorporating phase-change materials (PCMs) for passive thermal management absorbs excess heat during peak load conditions ensuring Active cooling controls should adjust cooling intensity based on real-time thermal data.
Safety and Redundancy
Systems should be designed with multiple layers of safety, including hardware and software-based protections. Incorporating and maintaining fail-safe mechanisms ensure vehicle control and safety during component failures. Equipping the vehicle with emergency isolation systems disconnects the battery during accidents or critical faults minimizing the risks.
Software Integration
Software plays a crucial role in managing EV systems. Developing software capable of real-time monitoring and diagnostics of all critical systems is essential. Enabling over-the-air (OTA) updates keeps the vehicle software current with the latest features and security patches. Implementing robust cybersecurity measures protects against potential hacking and unauthorized access.
Real-Time Monitoring
Real-time monitoring systems play a pivotal role in pre-emptively detecting and addressing issues within EV systems. Monitoring key parameters—such as Battery Health, Voltage, Current, and Temperature—in real-time allows for swift intervention in the event of anomalies, thereby averting potential system failures. Leveraging communication protocols like CAN bus, LIN bus, and Ethernet facilitates efficient data exchange for comprehensive monitoring and analysis.
Advanced Control Algorithms
The utilization of advanced control algorithms like BMS Algorithms, Power Electronics Control, Energy Management Systems, and Charging Control Algorithms among others represents another cornerstone of EV engineering. These algorithms govern power flow management, efficiency optimization, and load balancing, thereby enhancing overall system performance. Features like Maximum Power Point Tracking (MPPT) for solar-assisted EVs and regenerative braking systems exemplify the sophistication and efficacy of advanced control algorithms in modern EV design.
Future Trends in Automotive and Electrification
The future of Automotive Electrification in electric vehicle (EV) design is poised to witness significant advancements. From cutting-edge battery technologies to sophisticated power electronics and integrated vehicle systems, these innovations will drive the next generation of electric vehicles. As these technologies mature, they will not only enhance the performance and reliability of EVs but also make them more accessible and sustainable, paving the way for a cleaner and more efficient transportation ecosystem.
Advanced Battery Technologies
Future advancements are expected to focus on increasing energy density, reducing costs, and improving longevity. Key developments include solid-state batteries, which promise higher energy densities and improved safety by replacing liquid electrolytes with solid ones, leading to longer ranges and faster charging times. Additionally, silicon anode batteries, utilizing silicon in place of graphite anodes, can significantly increase capacity, offering more energy storage per unit weight. Improved battery recycling processes and repurposing batteries for secondary applications, such as energy storage systems, will also enhance sustainability.
Enhanced Battery Management Systems (BMS)
Future BMS will integrate more sophisticated algorithms and AI-driven analytics to optimize battery performance and lifespan. Enhancements include predictive maintenance, which utilizes machine learning to predict and prevent potential failures, reducing downtime and maintenance costs. Adaptive charging algorithms, employing smart charging strategies that adjust based on battery health, user habits, and grid conditions, will also maximize efficiency and lifespan.
Innovative Power Electronics
Advancements in power electronics are critical for improving the efficiency and reliability of power conversion in EVs. Future trends include wide bandgap semiconductors, such as silicon carbide (SiC) and gallium nitride (GaN), which offer superior efficiency and thermal performance compared to traditional silicon-based devices, enabling more compact and efficient power electronics. Additionally, the development of scalable and modular inverter designs will allow for easy adaptation to different vehicle platforms and power levels.
Integrated Vehicle Systems
The integration of various vehicle systems will play a crucial role in future EV design, enhancing overall efficiency and performance. Key areas include unified thermal management, which involves integrated systems that manage the thermal loads of batteries, power electronics, and the cabin environment more efficiently. Vehicle-to-everything (V2X) communication will enable EVs to communicate with the grid, infrastructure, and other vehicles to optimize energy use, traffic management, and safety.
Advanced Charging Solutions
Future EVs will benefit from faster, more convenient, and more efficient charging solutions. Key innovations include ultra-fast charging technologies capable of delivering significant power in a short time, reducing charging times to minutes rather than hours. Wireless charging systems will provide seamless and convenient energy transfer without the need for physical connectors. Furthermore, smart grid integration will optimize charging based on real-time grid conditions and renewable energy availability, supporting grid stability and reducing costs.
Autonomous Driving and Electrification
The convergence of autonomous driving technologies with EV electrification will drive new design considerations and opportunities. Autonomous systems can optimize driving patterns for energy efficiency, reducing overall consumption. Integration with dynamic wireless charging infrastructure will allow EVs to charge while in motion on specially equipped roads.
Conclusion
EVs are at the forefront of a transformative shift in the automotive electronics industry, heralding a new era of sustainable, efficient, and technologically advanced transportation. These systems, encompassing electric propulsion, battery technology, power electronics, and smart control mechanisms, are redefining vehicle performance and environmental impact. As technological advancements continue to enhance the capabilities and affordability of electric vehicles, they promise to address the pressing challenges of climate change, air pollution, and energy security.
The evolution of EVs and efficient Automotive and Electrification mechanisms not only represents a leap forward in automotive engineering but also signifies a commitment to a cleaner, greener future. Embracing these systems is crucial for driving innovation, fostering economic growth, and achieving a sustainable mobility ecosystem for generations to come. To know more about Mistral’s Automotive Electrification and Power Electronics offerings and related design services, please visit Automotive Electronics and Electrification or write to speak to a Technical Expert!